Electron Beam Metal 3D Printer (Additive Manufacturing Machine)
Electron Beam 3D Additive Manufacturing Technology
In recent years, 3D printers using resin based on digital 3D data have enabled
companies to produce prototypes and small-lot parts, and individuals to enjoy creating original,
one-of-a-kind products. 3D additive manufacturing machines using metal are one type of 3D printer. Most of
them are used for prototyping and manufacturing parts in the industrial field, and are rarely seen by the
general public.
The history of additive manufacturing technology is said to date back to the 1980s, when Mr. Kodama of the
Nagoya Municipal Industrial Technology Research Institute developed a lamination technology using a
UV-curing resin. Later, with its commercialization in the U.S. by Mr. Hull (now 3D Systems, Inc.), the
technology began spreading. The shift to metal additive (lamination) manufacturing began with the
development of a selective laser sintering method using metal powders, led by Deckard and Beaman at the
University of Texas in Austin, followed by its commercialization, and the development of a laser-based metal
additive (lamination)manufacturing system in 1992. This was followed by the development of a method using an
electron beam as a melting source by a Swedish company ArcamAB in 1997.
Up to now, the additive manufacturing has been utilized to confirm the shape and design and to prototype lightweight and strong parts. Recently, however, the short total development time required to produce a prototype and the ability to manufacture directly from digital data (CAD) have been attracting attention. Its use as a manufacturing method for industrial parts has been accelerating.
Principle of Additive Manufacturing
Here, we will mainly explain about the flow of molding parts with the metal 3D additive manufacturing machine using electron beams.
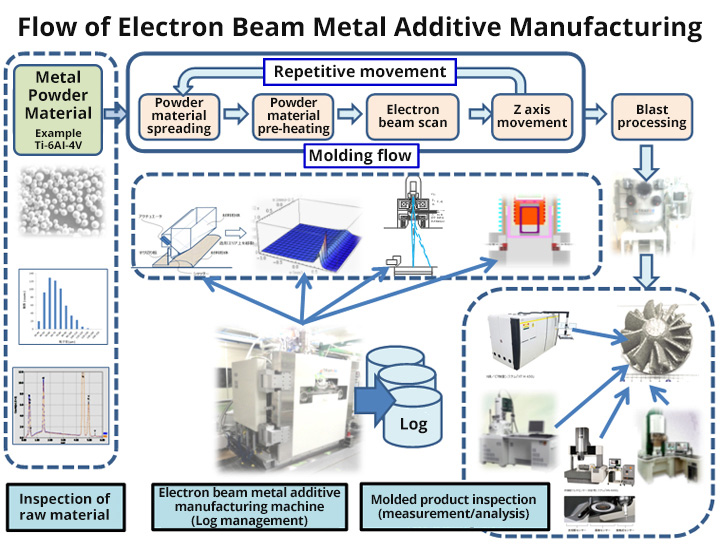
Figure 1 Flow of Electron Beam Metal Additive Manufacturing
The flow is to spread a layer of raw material of metal powder and melt the part to be molded by using a heat
source such as a laser beam or an electron beam and layer.
Continue layering and melting and once
completed, remove powders from the completely layered part for further quality testing.
The two methods are outlined here.
Powder Bed Method
The metal powder used as raw material is spread by a head mechanism capable of spreading it at a constant thickness.
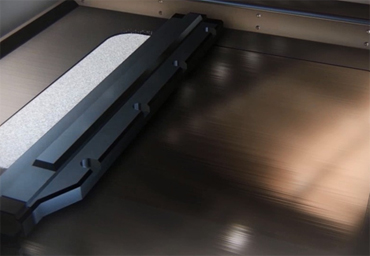
Figure 2 Movement to spread one layer of metal powders ※1
The first layer of material is spread and the area to be molded, which is created in the CAD data, is melted using an electron beam or laser as a heat source.
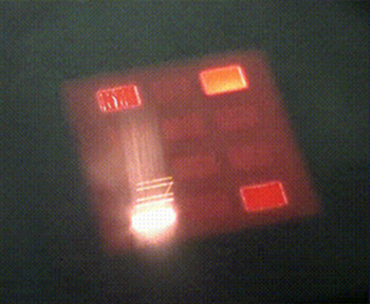
Figure 3 Melting of spread metal powder ※1
After moving the Z-axis by the thickness of the layer, the
second layer of metal powder is spread and the desired part is melted.
This process is repeated to form the desired shape.
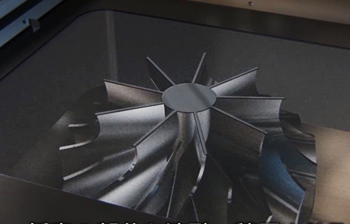
Figure 4 Image after removing unmelted powders ※1
The unmelted powders are removed by the blasting equipment,
etc. The molded part is taken out and inspected for shape and internal defects, etc.
The above process is how parts are molded.
Deposition Method
This is a method to layer powders by injecting powders through the nozzle to form a
desired shape designed by a CAD by moving a melting nozzle or stage. Metal powder materials are fed by using
a gas and melted by a heat source for molding.
Unlike the powder bed method, it is not required to remove powders after molding is completed. This method
is used for additional processing (weld overlay) to a metal material or molding of a general shape, rather
than for free form molding as the shape to be molded is limited by the reach of the head.
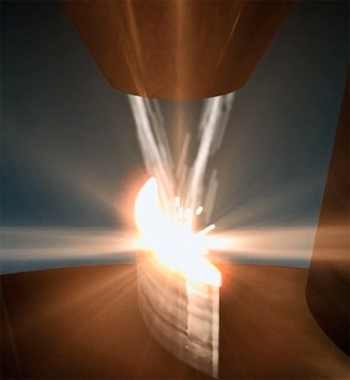
Figure 5. Deposition Method Case ※1
Feature of Additive Manufacturing
Because of its ability to directly melt and form materials from CAD design data, additive manufacturing is used for parts with high added value that require multiple prototypes and small quantities, strength design, design studies, and other production applications. Free-form molding is also being used to reduce material consumption through lightweight design and shape optimization, and to improve fuel efficiency by reducing weight.
Electron Beam Metal 3D Additive Manufacturing Machine
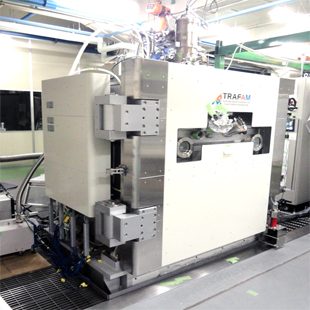
Photo 1 Example of an electron beam additive manufacturing machine
Photo 1 is the machine developed by JEOL Ltd. Akishima Branch Office ※1, Technology Research Association for Future Additive Manufacturing (TRAFAM) to realize the manufacturing function of different types.
The features are listed below.
- Additive manufacturing of different kinds of metals is possible.
- Minimum beam size: φ100 μm or less
- Accuracy: ±40 μ or less
- Moldable size: 300 mm x 300 mm x 600 mm
- Maintenance of the electron source environment by differential pumping, dynamically correcting stigmator, focus, and beam distortion correction function at the deflection position are employed.
Material and Molding
Since the electron beam type is suitable for molding of high-melting point metals, materials for industrial fields are used. Molding powders such as Ti-6Al-4V and Inconel718 are mainly used in the aerospace industry, while CoCr-based materials are used in the medical field due to their affinity as biomaterials. Spherical powders of several ten μms are used as metal powders. After they are manufactured by a gas atomizing or plasma rotating electrode process, the metal powders are selected by particle diameter distribution according to the additive manufacturing machine or the required quality of product to be molded.
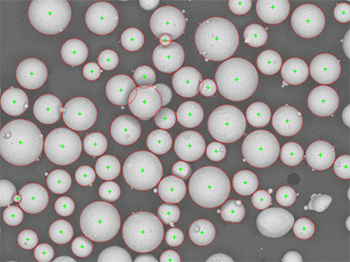
Photo 2. Raw material metal powder (Ti-6Al-4V) ※3
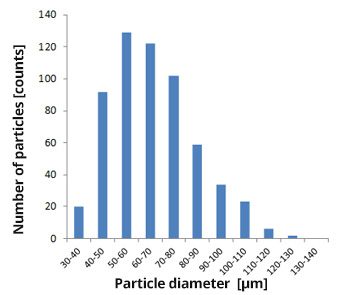
Figure 6 Particle diameter distribution example of material powder ※3
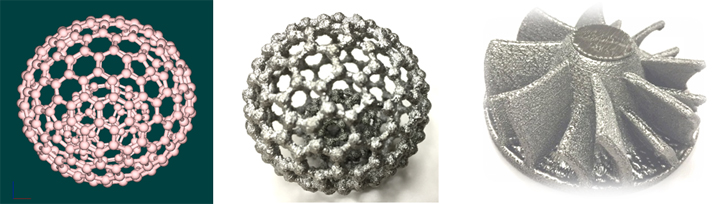
Photo 3 Examples of carbon fullerene dual structure, impeller ※1
Application for Electron Beam Additive Manufacturing
Electron beams used in additive manufacturing are utilized by generating a beam current of several mA to several hundred mA at an acceleration voltage of several tens of kV, which is necessary to melt the metal powder. The electron beam is a flux of electrons and once collided with metal powder, the kinetic energy becomes vibrational energy of the target lattice and is converted into heat. While light beams generate reflection and the conversion efficiency differs according to the material, the heat conversion efficiency of the electron beam is extremely high, with more than 80% of the input energy being converted into heat. In order to irradiate the electron beam to the desired position provided by digital data, performing deflection by using a magnetic lens enables high-speed scanning because it is electrical rather than mechanical scanning.
Electron Source for Additive Manufacturing
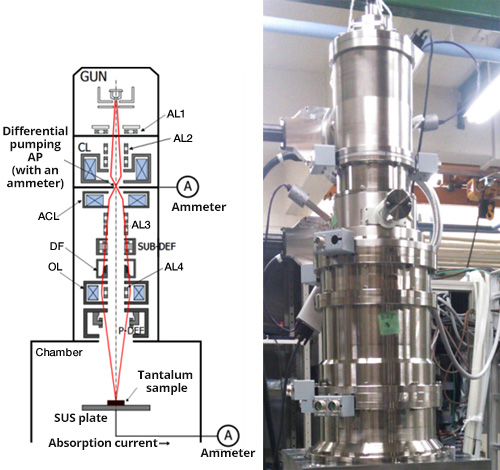
Figure 7 Electron beam irradiation system ※1
An electron gun generates electron beams, controls the beam shape and irradiation position, and irradiates the electron beam to the target object for processing. The structure of a typical electron beam irradiation system is shown in Figure 7. It consists of an electron beam generator, an electron beam converging section, and an electron beam deflector.
Advantage of using Electron Beam as Heat Source
- Processing in vacuum
Electron beams are generated, propagated, and irradiated in a vacuum. In the metal melting process, this has the effect of reducing inclusion of impurities into the melting section, resulting in higher quality materials. - High-speed scanning
As the irradiation position of the electron beam is controlled by using an electromagnetic coil, extremely high-speed scanning is possible on the processing target surface. With the electron gun that is used in metal additive manufacturing, it is possible to scan electron beams at a speed of several 1,000 m/s or higher. - Highly efficient transmission
Since there are no optical parts that can cause energy losses between the cathode, which is the generation source and the processing target, electron beams are not affected by the reflection rate and transmission rate. Thus, precise control of irradiation energy is possible for the processing target. - High absorption rate
Because the electron beam is a high-speed stream of electrons, most of it is absorbed into the processing target without being reflected, and converted into heat of fusion.
Quality Assessment of Additive Manufacturing Product
The discussion is on-going about the international standardization for the standard molding testing piece and its evaluation in metal additive manufacturing, by the technical committee ISO/TC261 and ASTM committee F42 on additive manufacturing technologies. The evaluation of moldings requires a wide range of measurement techniques from standard test pieces to inspection of moldings to maintain quality. This section describes typical conventional standards and effective evaluation and analysis methods for additive manufacturing methods.
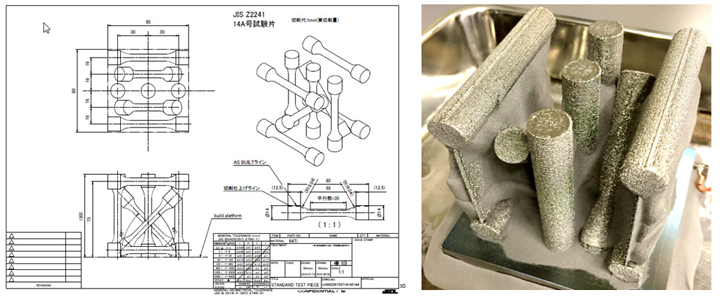
Photo 4 JIS test piece molding for additive manufacturing (before cutting) ※1
Shape Measuring Method
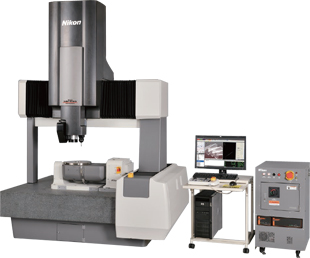
Photo 5 3D shape measuring instrument ※2
① 3D shape measuring instrument
Measurement is classified into the mechanical contact method and non-contact method. The contact type is based on point and line measurements using a touch probe and rotates the object to form the shape. On the other hand, the non-contact method uses laser irradiation and an optical camera to scan the beam for high-speed measurement. Both methods have a measurement accuracy of several μm to several tens of μm.
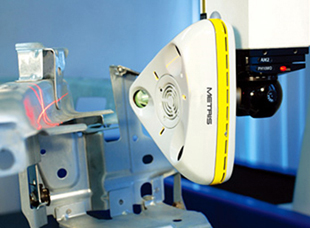
Photo 6. 3D shape measurement instrument ※2
② 3D scanner
Similar to taking measurements, there are contact and non-contact methods. As free-form shapes are often measured in additive manufacturing, use of a non-contact laser or infrared light for shape measurement is the mainstream. The accuracy is several tens μms and faster 3D measurement is possible than the contact method.
Tissue Observation
Scanning electron microscopes (SEM) and surface analyzers (EPMA) are capable of not
only surface observation of materials but also elemental analysis and crystal orientation observation using
onboard detectors. They are widely and commonly used from non-destructive inspection of surface to
destructive inspection by cutting cross sections.
When a focused electron beam is irradiated to a
material to be observed, information on the quantum generation, such as secondary electrons in the depth
direction, is obtained depending on the element. Analysis is possible by using a detector that converts
these generated quanta into signals.
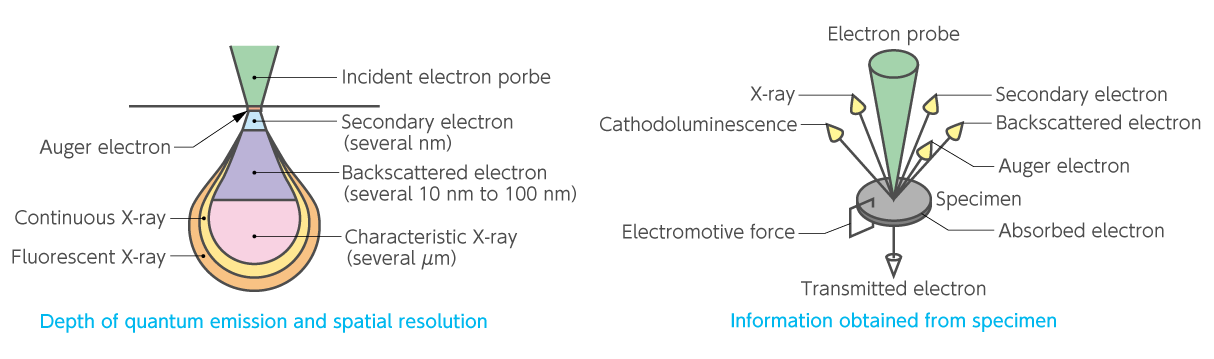
Figure 8 Electron beam and quantum types
Secondary Electron Image Observation | Secondary electrons are used to observe the topography of the specimen surface. |
---|---|
Backscattered Electron Image Observation |
Backscattered electrons are mainly used to observe the compositional differences of the
specimen. In the case of a polycrystalline specimen, the differences in crystal orientation are observed as the contrast in the image. (channeling contrast) |
Absorption Electron Image Observation | Absorbed electrons are mainly used to observe the compositional differences of the specimen. The contrast is the reverse of that for the backscattered electron image. |
Transmission Electron Image Observation | Electrons transmitted through a thin film layer are used to observe the differences in composition and density. |
Cathodoluminescence(CL) Image Observation and Spectrum Analysis | The light emitted from a specimen stimulated by electron beam irradiation forms an image, and an emission spectrum is created by means of wavelength spectrometry. This is used for applications such as the evaluation of impurities and defects in semiconductors, the evaluation of stress distribution, the evaluation of defect structure distribution in the oxide film, the evaluation of light emitting elements, etc. |
EDS(Elemental)Analysis | This is a function to obtain a spectrum of the energy intensity of X-rays using a combination of the Li- doped Si semiconductor detector and the Multi-Channel Analyzer (spectrum analyzer). All elements from B to U can be detected and measured simultaneously. Even with a modest probe current, which decreases the risk of specimen damage,it performs excellently in the micro area analysis. |
EBSD Analysis | Backscattered electrons which have been diffracted on a specimen surface are used to identify crystal orientation in a micro area and to measure the orientation map. |
WDS(Elemental) Analysis | This is a function to obtain a wavelength spectrum by utilizing the diffraction phenomenon of an X-ray by a crystal. Its features are high energy resolution and high detection sensitivity. |
EBIC Measurement, Observation | The electromotive force (EMF) generated inside a specimen due to irradiation by an electron beam is used for defect analysis of semiconductor devices. |
Low Vacuum Function |
A function to set the pressure in the specimen chamber to several tens to several hundred Pa. Decreasing the vacuum in the specimen chamber reduces the occurrence of charging. This makes it possible to observe a non-conductive specimen without the need for a difficult pre-treatment (coating). It is used for specimens with a lot of outgassing, specimens with a low vapor pressure, and specimens containing water as well. |
Electron Beam Exposure Function | Resist lithography by electron beam is possible. |
Cryo SEM Observation | Observation of a water-containing specimen is possible by freezing the water. This can prevent deformation of a specimen from occurring during the processes of fixation and dehydration. |
Heating Observation |
Observation while heating a specimen is possible. Changes occurring to a specimen caused by heat such as swelling and segregation of impurities can be observed. |
Tensile Observation | Observation while stretching a specimen is possible. This is used to observe the starting point of ductile fractures and analysis of material strength. |
Table 1 Information obtainable from analyzed samples
Here is the result of EDS elemental analysis of Ti-6Al-4V powder that is used for additive manufacturing machine to confirm the components of Ti, Al, and V.
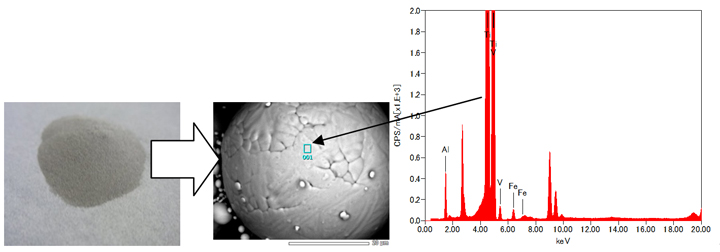
Figure 9 Observation of Ti-6Al-4V molding powder and analysis example by X-ray fluorescence spectrometer (JSX-1000S of JEOL) ※3
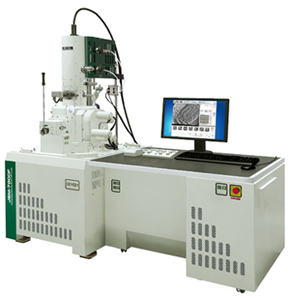
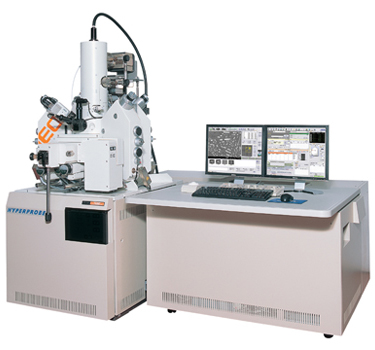
Photo 8 Appearance of electron microscopes
A transmission electron microscope (TEM) is used for atomic level observation. Electrons are unable to freely move in the air, but are in a vacuum. Unlike optical microscopes, an electron microscope maintains a high vacuum in its column to help electrons move. The electrons emitted from the electron source called the electron gun are accelerated and irradiated to the irradiation surface. Electrons transmit a specimen or scatter. The electrons are converged or scattered by using an electron lens (electric field of magnetic field) to form an image. Conventionally, the image was enlarged on the fluorescent plate and observed by human eyes. But now the mainstream is to convert it into an electrical signal by using image sensors such as CCD and display as a digital screen. The light that human eyes can distinguish (visible light) is 400 nm to 800 nm. Transmission electron microscopes generally use high voltage of more than 100 kV accelerating voltage. The wavelength of 100 kV equals to 0.0037 nm, which indicates a resolution, meaning that it has an adequate resolution to observe an atom of several nm.
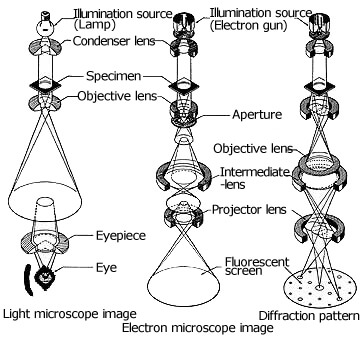
Figure 10 Transmission Electron Microscope Principles
Non-destructive Test
As a non-destructive inspection method for defects and voids using images, X-ray CT
can be used to inspect free-form products in additive manufacturing. The X-ray CT enables measurement of the
sample in 5 axes and detection of the defect positions by 3D display.
The resolution and transmission capability depend on the output of the microfocus X-ray source, but
generally 150 kV to 45 kV is used. Resolution ranges from a few micrometers to several hundred micrometers,
depending on the size of the measurement and the transmittance of the material. While higher output improves
resolution, it also requires countermeasures against X-ray leakage, and these countermeasures are more
extensive.
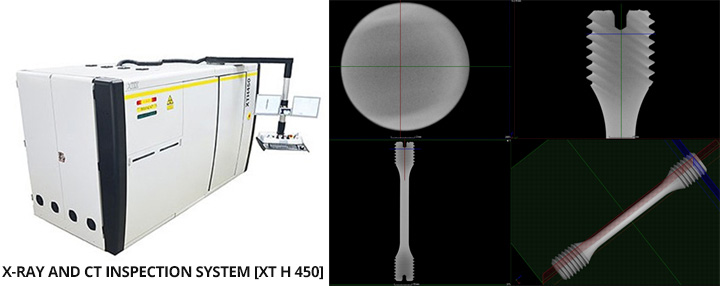
Photo 9 JIS A2201 14A test specimen observation case ※2
Efforts are being made to improve quality by classifying defects observed without destruction and contrasting them with what caused the defect during additive manufacturing. The need for non-destructive inspection of quality-critical industrial parts is expected to increase in the future.
Conclusion
The 3D additive manufacturing using metal is attracting attention as a method to
produce a desired part directly from digital data. In addition, by using the molding capability of free-form
which is a feature of 3D molding, its use is considered to expand in the future for optimal strength design
and as an energy-saving manufacturing method.
As it does not require an extensive facility, it is also being considered as a part of a distributed
manufacturing method that assumes network connectivity of the manufacturing equipment in the IoT era.
JEOL supports the advancement of technologies by integrating the additive manufacturing technology that may
trigger a new innovation, with the measurement and analytical technologies to maintain the product
quality.
The results were achieved through a project commissioned by the Ministry of Economy, Trade and Industry
(METI), "Development of the next-generation industrial 3D printer technology and ultra-precise 3D molding
system technology". We would like to thank the following organizations for their support during our
research.
- Ministry of Economy, Trade and Industry
- National Institute of Advanced Industrial Science and Technology (AIST)
- Specially Appointed Professor. Hideki Kyogoku, Engineering Department, KINDAI University
- Akihiko Chiba, Professor, Institute for Materials Research (then), Tohoku University
- Technology Research Association for Future Additive Manufacturing (TRAFAM)
Reference
-
Material provided by the Technology Research Association for Future Additive Manufacturing (TRAFAM) which conducts "Development of the next-generation industrial 3D printer technology and development of an ultra-precise 3D molding system technology" commissioned by the Ministry of Economy, Trade, and Industry.
https://trafam.or.jp/top/ -
Materials provided by Nikon Metrology Inc. (web site)
https://industry.nikon.com/en-us/ -
Materials provided by LPW Technology Ltd.(then)/Aichi Sangyo Co., Ltd.
-
Please refrain from reprinting or diverting this material.